Laser Induced Breakdown Spectroscopy (LIBS)
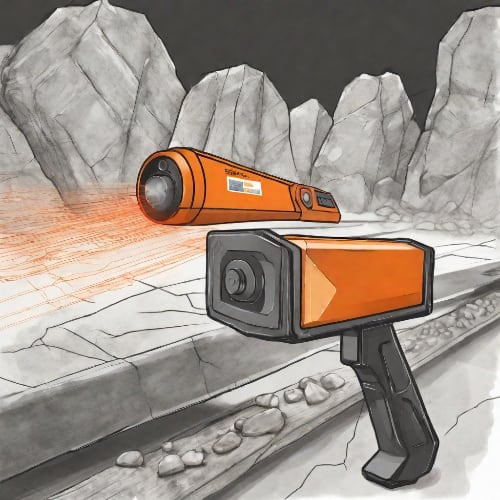
LIBS stands for laser-induced breakdown spectroscopy and is a rapid chemical analysis technique, first introduced in 1960s. Unlike in Raman spectroscopy, it uses short laser pulses to create plasma plumes on the surface of a sample and the spectrum is characterized of this plasma using low-cost spectrometers. It is also categorized as an in situ operation, that’s duration can last up to only a few seconds.
How It Works
The whole process can be separated into few distinguished parts: laser ablation, plasma formation and the cooling of the ignited plasma. The focused laser pulse initiates a high temperature raise, generation of free-electrons. During this process a part of the sample mass is removed, the process is called laser ablation. Afterwards, the ablated mass interacts with the rest of the laser pulse, and microplasma is formed. It contains free electrons, excited atoms and ions. Such excited particles are formed, when the laser-emitted photons are absorbed by the sample atoms and molecules, allowing them to reach excited energy levels. As a result plasma is formed at the surface of the sample. When the laser pulse ends, the plasma begins to expand and cool. The energy is then lost in the process of recombination. Later on, the emitted light is collected and analyzed by a high-speed camera, detector or a sensor, the most popular one being Czerny-Turner and Echelle spectrographs. The secondary light of the plasma is collected and transported through an optical fiber to the detector (Figure 1). Spectroscopy principles can be applied to this operation, since each element or material has a defined peak intensity wavelength that it emits. A specific element has its own LIBS spectral peak fingerprint, that can be used to identify the existing elements in the sample. The received spectra are defined by wavelength, intensity and the shape of the signal. This intensity information on the received spectra peaks can define the quantity and concentration of a specific element in the chemical composition.>
Laser Induced Photoablation
Photoablation, or laser ablation is defined as a process, in which a miniscule amount of the surface material (solid or liquid) is removed off of a sample. The high amount of energy leads to the evaporation or sublimation of a material, that is induced due to the received energy of the absorbed photons. The energy amount is so great, that the material is converted to plasma state.
The most important part of the whole LIBS system and the main tool to attain the ablation effect is a pulsed laser. Both a femtosecond and a picosecond laser can be utilized, though the nanosecond laser is recalled as the most preferable one. Most commonly, a pulsed fundamental harmonics (1064 nm or 1030 nm wavelength) laser is used, as well as its harmonic wavelengths (532 (515), 355 (343), 266 (257) nm). All of these selected devices create different effects on the sample material, but when a nanosecond laser is used, the main driving force of the ionization process are thermal effects. Since the duration of the course of heating is about 104 times shorter that the laser pulse itself, for a brief moment, a part of the sample is melted and vaporized. It is worth mentioning, that both a free-space and a fiber laser can be used, both are a great light source for LIBS setups.
The properties of the selected laser tightly correspond with the absorption of energy, since the received energy depends on the emitted photon characteristics. These parameters include the lasers wavelength, pulse duration and fluence. For the reason of energy being reversely dependent on wavelength, a shorter wavelength induces a greater ablation rate, though a higher wavelength can result in a more efficient plasma excitation. Another, already mentioned, parameter is the pulse duration - it determines the effects taking place before the plasma is created. When nanosecond pulses are selected, the material undergoes thermal processes and changes from solid, through liquid and gas, to plasma, while the rest of the pulse heats the plasma (the plume itself can reach up to 15000-30000 K temperature). Pulses of nanosecond duration are favored, since they heat up the microplasma at a slower rate, as a result a larger and less dense plume is generated. This leads to clear and intense emission lines, that make the data reading process much more easy and smooth. Lastly, the intensity and fluence (energy per area) of the laser pulse have to reach a certain threshold (ionization potential) to produce sublimation, evaporation and the formation of the high-density plasma. A quite similar characteristic is irradiance (power per surface area) - it must be optimized, for the reason of most effectively ablating the smallest speck possible. This can be achieved by combining a lens with a high irradiance laser source. Certainly, other, non-laser related, environmental factors and properties of the sample material play a role in the whole LIBS process, though is it the laser source that is readjusted accordingly to the given circumstances. As all the mentioned properties play a significant part of the laser ablation process, it is the delicate combination of all, that produces the desired effect.
Plasma Lifetime
The life time of the plasma plume has been observed in depth, its evolution and effects occurring during it have been described properly. Several states in the evolution of plasma can be separated, such including formation, shielding, cooling and emission radiation.
The plasma itself is created after the photoablation process. After the plasma creation, during a following time period called plasma shielding, the plasma becomes opaque to the laser, and the photon pulses begin to interact only with the surface of the created plasma plume. The latter reflects the laser energy away from the rest of the sample, reducing the overall ablation rate. During this time period, the microplasma is heated up and expands, thus sending shock waves through the environment, as the surrounding particles are compressed. The remaining time is dominated by the process of cooling and spectrum emission. This happens through recombination of electrons and positive ions and de-excitation of atoms, ions and molecules. The excited particles begin to lose their kinetic energy in the form of light, with their characteristics, relevant to the chemical structure of the sample.
During the lifetime of the plasma, few types of radiation can be observed, including continuum, atomic, ionic and molecular emissions, all of them revealing different plasma components. The first moments of the ignited plasma plume are dominated by continuum radiation. The emission that shows the relevant information is the atomic radiation, which happens right after the plasma has cooled. The received spectra are described by their wavelength, shape and intensity. The wavelength of the received signal helps to deduce the energy levels of the sample material, while the different intensity of atomic lines indicate the quantity of the specific element. The collected data greatly depends on the mass that was ablated, the received energy from the laser and environmental conditions.
In the process of LIBS, it is highly important to carefully choose the gate time interval, the exact moment when it begins and ends, due to the fact that plasma creates delays the atomic lines emission window. The delay time and integration time are both defined by the wavelength of the laser utilized. Both of these time periods are analyzed in relation to one another – by experimentally comparing the delay and integration time, a signal to noise ratio is observed. Often the integration time is referred to as a gate window, this exact time period provides the needed information for trace spectroscopic analysis.
Figure 1. Simplified diagram showing the LIBS process.
Applications
The described technique is a valuable tool for research of delicate or fragile objects or materials. Numerous examples can be listed, one of them being the field of archeology, where LIBS proves itself to be a practical way to date certain samples, such as dyed artifacts, sculptures, ceramics. The method is utilized in biomedicine – it can perform as a tool to analyze and classify various tissues, detect and treat tumors. In the industrial field, it is a common technique used on different products, to specify their chemical composition, like determine dopants in dielectrics, sort recyclable metals or plastics. LIBS has also been used for measurements including dangerous materials, such as radioactive and toxic substances or explosives. It is possible to detect the same toxic materials or waste in geological samples, as well as monitor selected substances in a specific environment (e. g. pollution in water or air). Such environmental analysis can be carried out by adapting LIBS, even so, that these devices are being used for space exploration, like in the Martian mission – the rover Curriosity contains a LIBS instrument ChemCam, which is used for chemical composition analysis of rocks and soil of Mars. Indisputably, laser induced breakdown spectroscopy is a widely applicable analysis technique.
Some advantages that LIBS carries with itself are worth mentioning - no preparation is needed for the sample, the short measurement time (a few seconds for a specific spot of the specimen), the technique can cover a broad variety of elements, can include surface of depth profiling by using different sampling protocols. In all cases, LIBS has been described as a beneficial chemical analysis technique, with a gradually growing development and usage.
Additional Literature
F. Anabitarte, Laser-Induced Breakdown Spectroscopy: Fundamentals, Applications, and Challenges, 2012 (OPEN)
Francisco J, Fortes, Laser Induced Breakdown Spectroscopy, 2012
L. Jolivet, Review of the recent advances and applications of LIBS-based imaging, 2018
Relevant Products
1030 nm Nanosecond Q-Switch Laser
Spectral line width FWHM, nm: 0.7
Pulse energy, µJ: 100
Pulse-to-pulse stability, %: 20
Warranty, months: 14 (Limited)
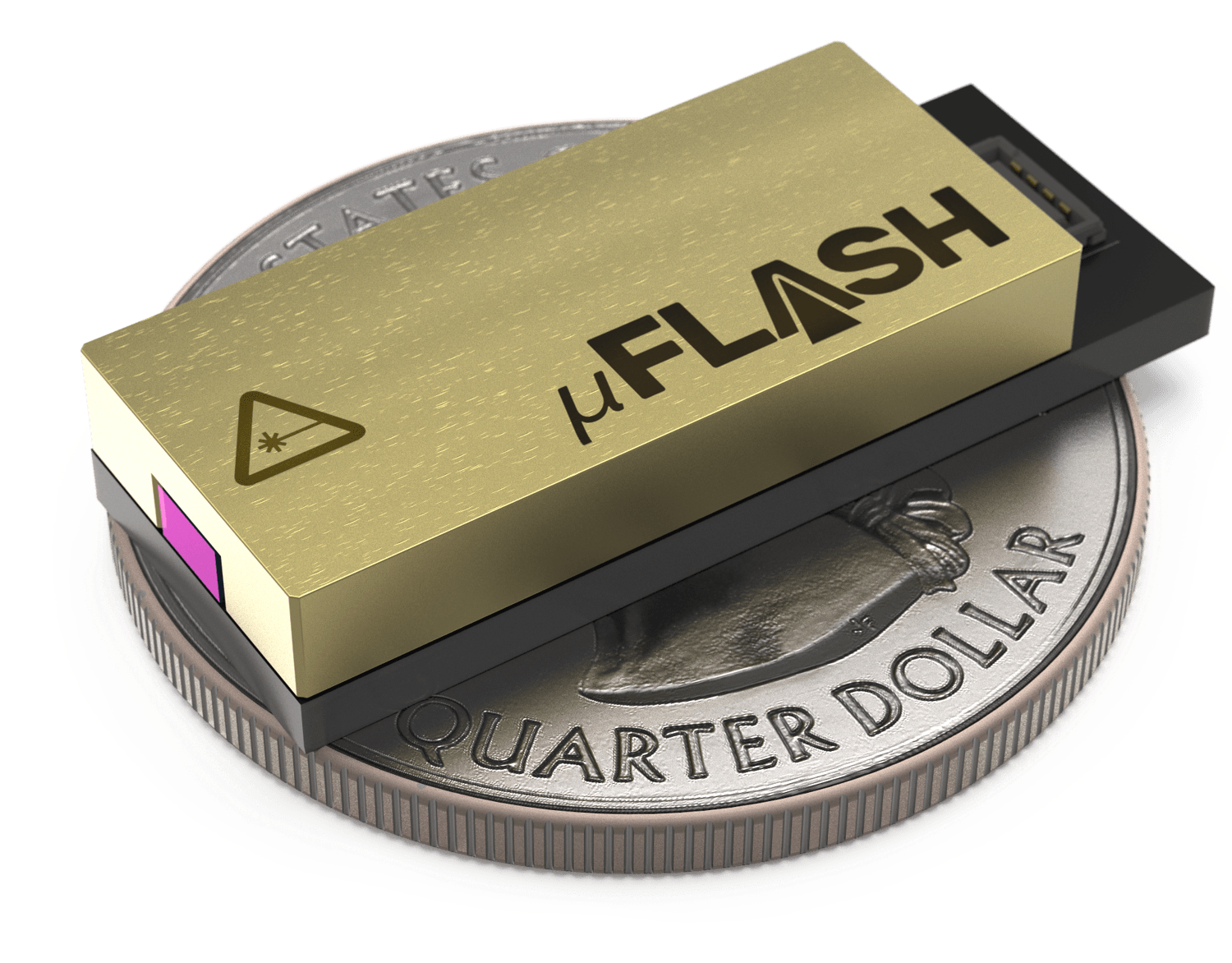
1030 nm Laser
Spectral line width FWHM, nm: 0.7
Output power, mW: As per request
Power stability, % (RMS, 8 hrs): 0.5
Pulse-to-pulse stability (RMS of peaks): 10
Warranty, months (op. hrs): 14 (10000)
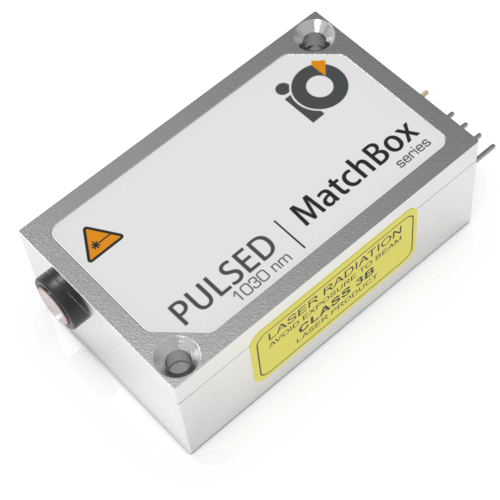
1030 nm SLM Laser
Spectral line width FWHM, pm: 0.8
Power stability, % (RMS, 8 hrs): 0.5
Pulse-to-pulse stability, % RMS: 2
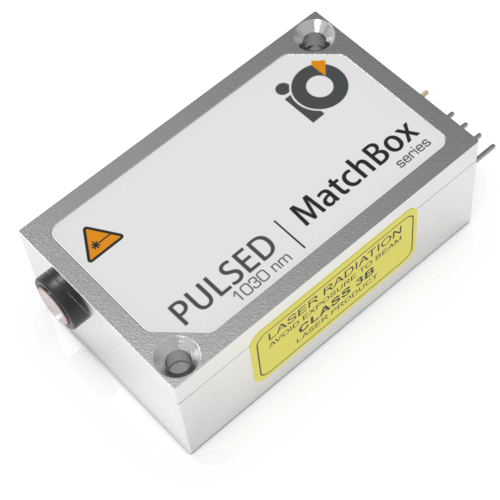
Publications
EarthDoc: