Fluorescence Lifetime Decay
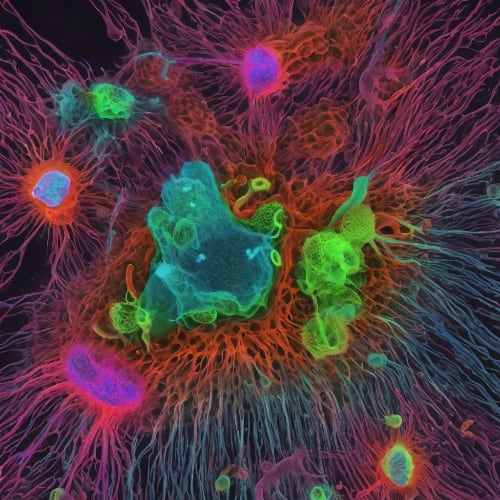
Fluorescence lifetime decay is the process of measuring how long a fluorescent molecule stays in its excited state before returning to its ground state. It can provide information about the molecular environment and interactions of the fluorescent molecule, as well as its structure and dynamics.
The principle of fluorescence is essential to grasp the analytic power of Fluorescence Lifetime Decay (FLD) as a scientific tool. That principle has been discussed in our article “Fluorescence Spectroscopy”. Here, we delve into the fundamentals of FLD, its compatibility with diode laser technology, and relevant applications.
Theory Behind Fluorescence Lifetime Decay:
FLD is the measurement of the time it takes for a fluorophore to transition from an excited state to the ground state. In other words, it is the duration between excitation and fluorescence, which typically lasts on the order of nanoseconds (as the duration of internal conversion is negligible compared to fluorescence). The actual time is highly dependent on the molecular configuration of the fluorophore, its polarity, temperature, and the presence of fluorescence quenchers. While fluorescence and decay occur on a per-molecule basis, it is the population behaviour that is observed in a sample, from which the fluorophore's properties are analysed. Therefore, the duration of this process and comparison between known values can provide valuable information about protein-protein binding, enzyme activity, and conformational changes.
Quantifying Excited State Decay in a Population:
Because fluorescence is an optical phenomena resulting in emission of a photon, intensity is a valuable metric to quantify and define ‘lifetime’. In the context of a population of fluorophores, fluorescence lifetime is typically defined as the duration it took for the intensity to drop to ~37% (an expression known as 1/e) of its original value (Io) (Figure 1.). This can be expressed in the relationship:
I(t)=Ioe-t/τ
where I(t) is the intensity dependence on time and and τ is the average time a molecule takes to transition from an excited state to the ground state. It can be approximated as:
τ=1/(kr+Σknr)
where kr is the transition rate constant and knr is the non-radiative constant. These constants can be obtained both experimentally and theoretically.
Figure 1. Diagram showing the typical, exponential, fluorescence intensity decay after laser pulse excitation.
Diode Lasers in FLD Imaging Systems:
There are 2 qualities that make diode lasers particularly suited for techniques incorporating FLD.
Firstly, diode lasers can generate a beam containing a single longitudinal mode (SLM), meaning light of only a very narrow wavelength range will stimulate the fluorophore. This quality provides high specificity, especially in systems containing multiple fluorophores, some of which have overlap in their excitation spectra. The inverse relationship between the photon’s frequency/wavelength and energy carried, implies that photons of specific frequency ('colour') will closely match the energy gap between S0 and S1 of a fluorophore. That wavelength of light is best suited to excite them and lead to fluorescence. Because SLM lasers have a particularly narrow distribution of photon frequencies emitted, they are great choices for integration into systems designed for highest specificity.
Secondly, in FLD Microscopy, excitation techniques often utilise short bursts/pulses of light, to briefly excite the fluorophore and measure the elapsed time. In such cases, diode lasers are particularly relevant due to their capability for rapid on-off modulation rates, some reaching up to 100MHz. These high modulation rates allow for the capture of fast fluorescence decay events, providing a detailed picture of the dynamic processes within the sample. Moreover, pulse shaping, which refers to controlling the duration of the ‘on’ time in a single pulse, allows for tailored excitation durations depending on the specific fluorophore. Lastly, rapid modulation enables the system to capture multiple fluorescence events within a single imaging frame; within a single imaging frame (which consists of many pulses), multiple fluorescence events can be recorded. This increases the overall efficiency and speed of data acquisition, allowing for precise quantisation of events in both the spatial and temporal domains. Because of this, diode lasers an excellent choice for techniques requiring high temporal resolution, such as Fluorescence Lifetime Imaging Microscopy (FLIM).
Incorporating Time-Correlated Single-Photon Counting (TCSPC) in FLD Studies:
Time-Correlated Single-Photon Counting (TCSPC) is a powerful technique that can be used to measure the Fluorescence Lifetime Decay (FLD) in Fluorescence Lifetime Imaging (FLIM). It is particularly useful for understanding the dynamics and interactions of fluorescent molecules. In TCSPC, a fast detector is used. It is synchronized with the excitation source (a laser), allowing it to measure the time delay between the excitation pulse and the arrival of the emitted photon with high precision (precise enough to count picoseconds). TCSPC data is statistical in nature. Each photon contributes to a histogram of photon arrival times, which can be analyzed to extract the fluorescence lifetime. This analysis typically involves fitting the decay curve to an exponential function, as the fluorescence intensity is expected to decay exponentially with time (Figure 1). The rate of this decay is determined by the fluorescence lifetime.
Fluorescence Lifetime Imaging (FLIM) and its Relation to FLD:
Fluorescence Lifetime Imaging (FLIM) is a technique that uses the fluorescence lifetime as a contrast mechanism to create an image. In the context of FLIM, TCSPC can provide a detailed map of the fluorescence lifetime across an image, providing valuable information about the molecular environment and interactions of the fluorescent molecules. This can be used to study protein-protein binding, enzyme activity, and conformational changes, among other things. Therefore, the combination of FLIM and TCSPC offers a highly specific and sensitive method for studying molecular processes.
FLIM works by exciting a sample with a pulsed light source and then measuring the fluorescence lifetime at each point in the image. The fluorescence lifetime is a unique property of each fluorophore, and it can be influenced by the local environment, such as the pH, temperature, or the presence of other molecules. By combining FLIM and TCSPC it is possible to obtain high resolution in the spatial and temporal domains.
Relevant Products
405 nm SLM Laser
Spectral line width FWHM, MHz: 20
Output power, mW: 50
Power stability, % (RMS, 8 hrs): 0.05
Intensity noise, % (RMS, 20 Hz to 20 MHz): 0.2
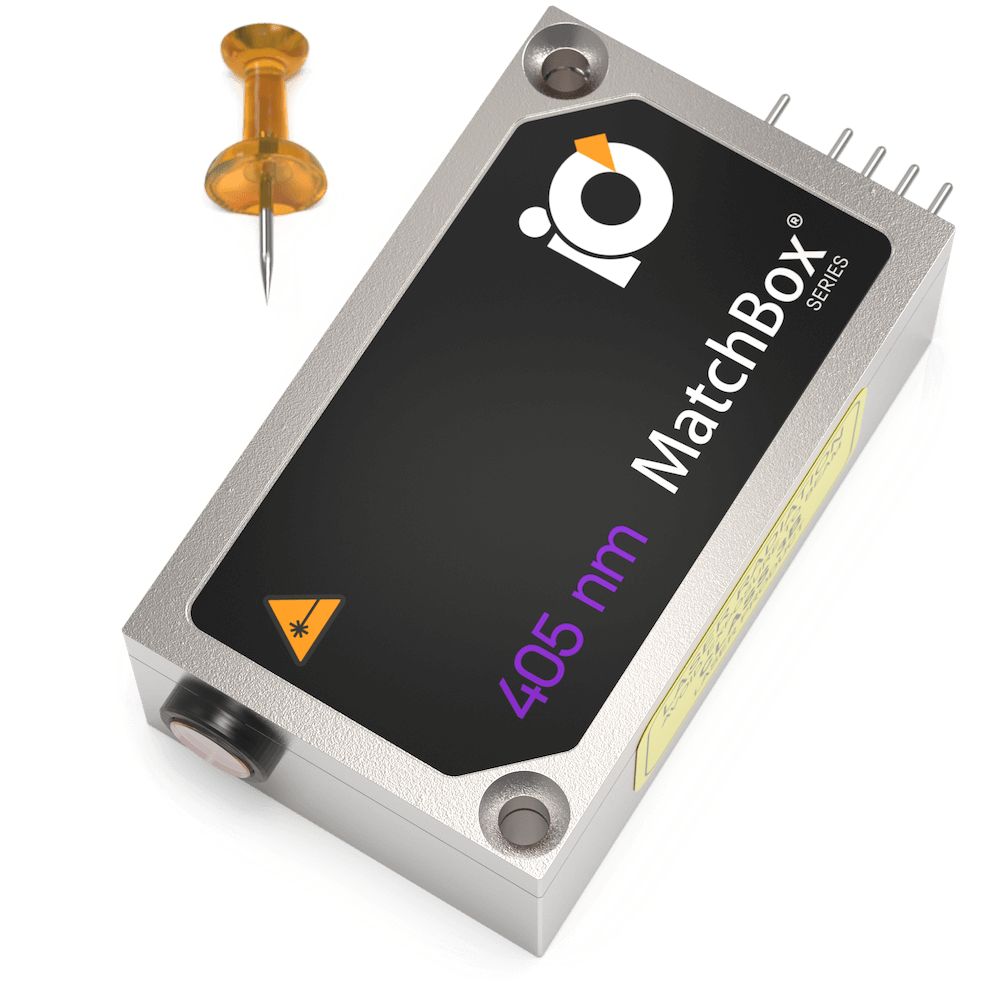
660 nm Laser
Spectral line width FWHM, nm: 0.7
Output power, mW: 110
Power stability, % (RMS, 8 hrs): 0.05
Intensity noise, % (RMS, 20 Hz to 20 MHz): 0.2
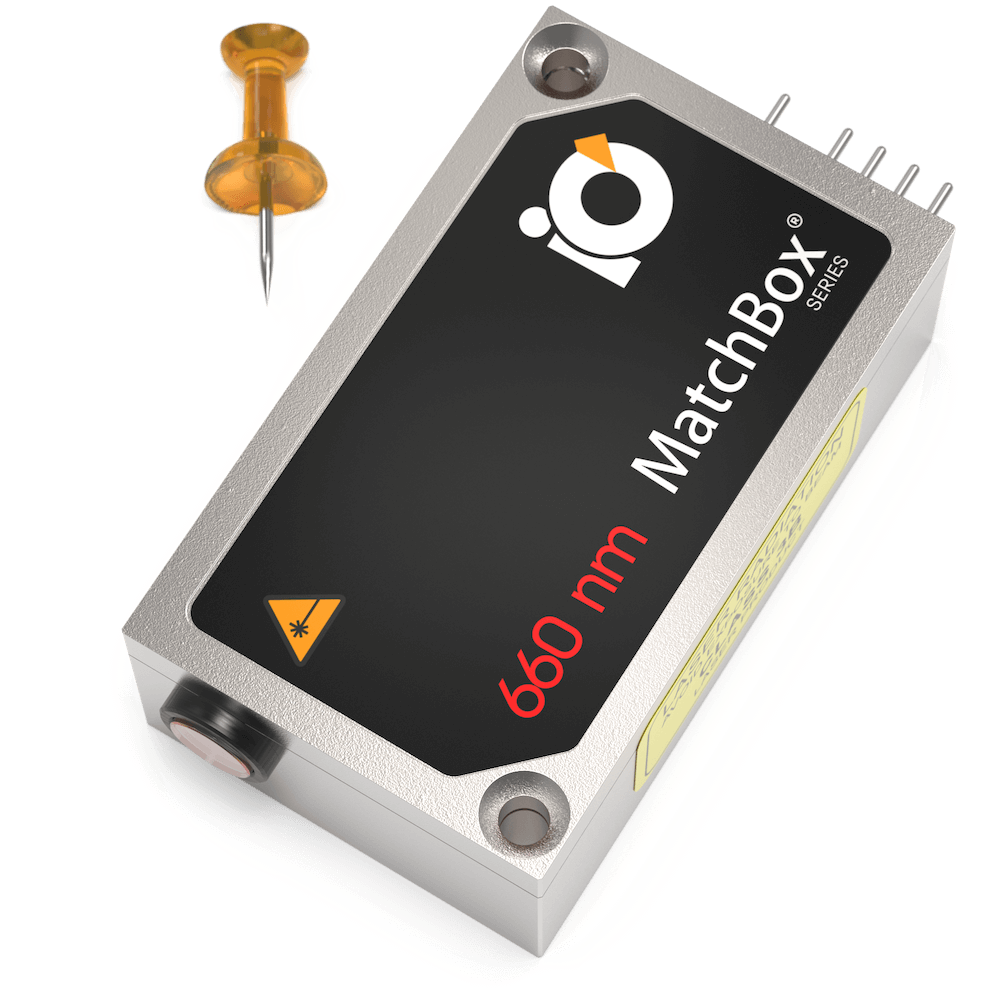
633 nm SLM Laser
Spectral line width FWHM, MHz: 2
Output power, mW: 70
Power stability, % (RMS, 8 hrs): 0.03
Intensity noise, % (RMS, 20 Hz to 20 MHz): 0.2
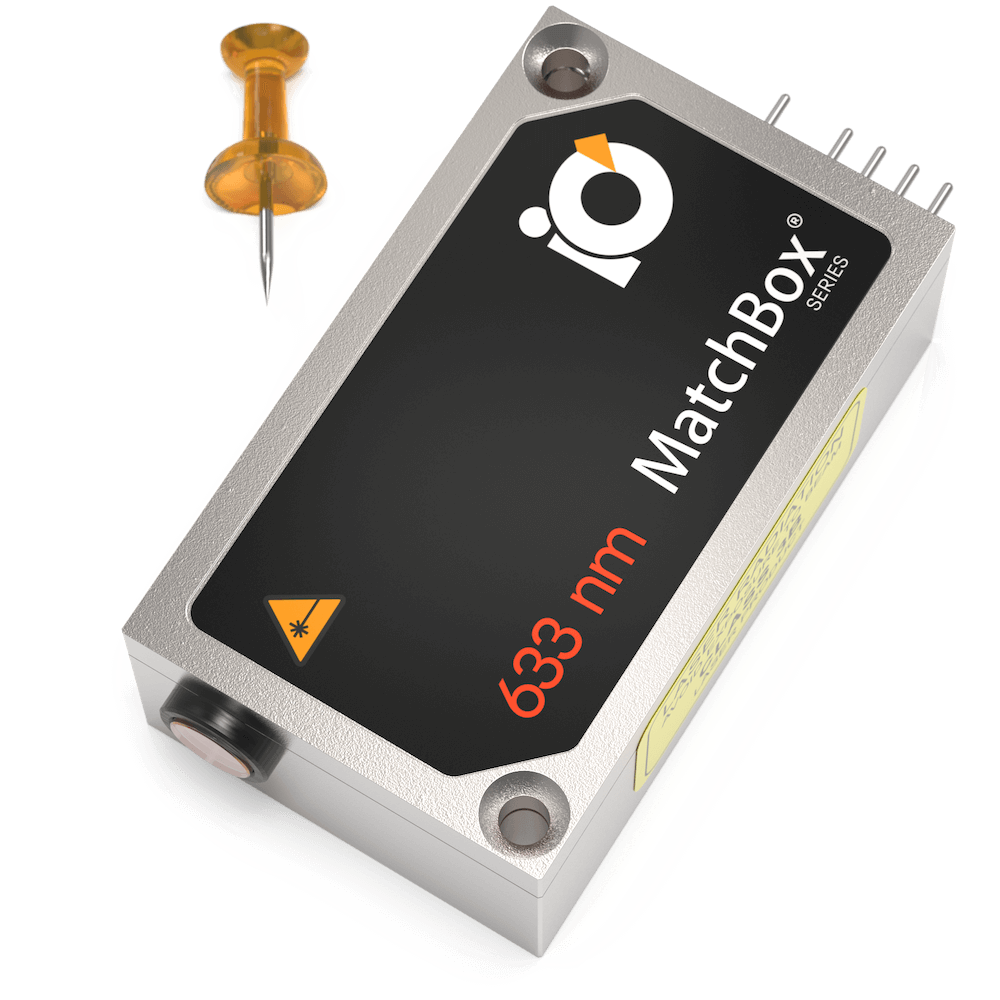
638 nm SLM Laser
Spectral line width FWHM, MHz: 2
Output power, mW: 100
Power stability, % (RMS, 8 hrs): 0.03
Intensity noise, % (RMS, 20 Hz to 20 MHz): 0.25
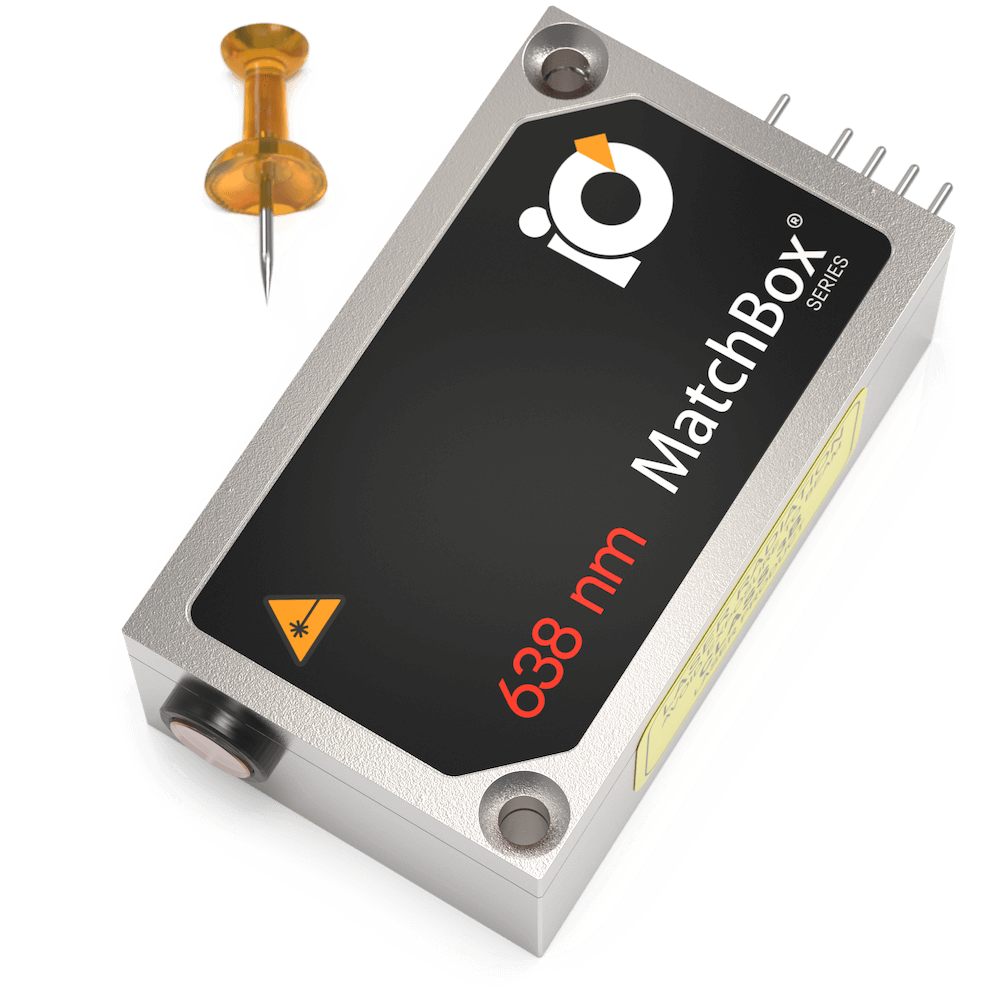
505 nm Laser
Spectral line width FWHM, nm: 0.7
Output power, mW: 60
Power stability, % (RMS, 8 hrs): 0.03
Intensity noise, % (RMS, 20 Hz to 20 MHz): 0.5
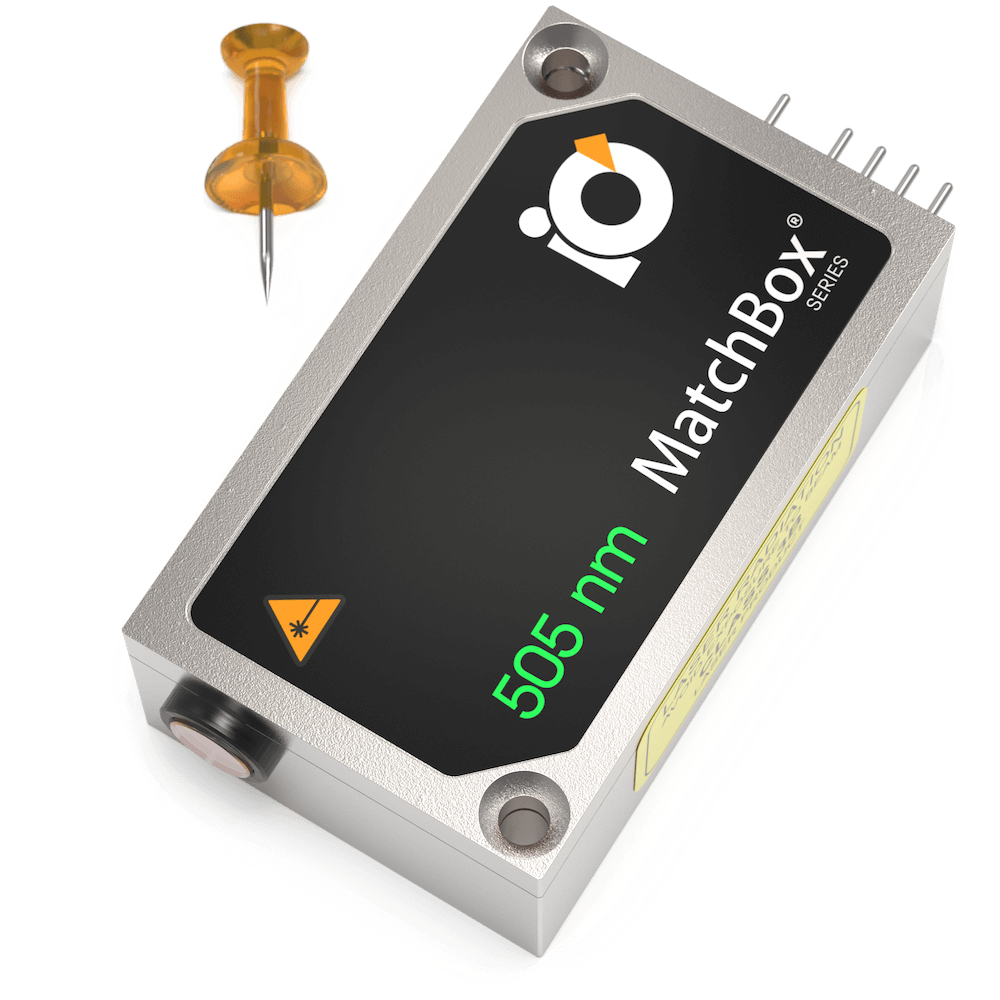
450 nm Laser
Spectral line width FWHM, nm: 0.8
Output power, mW: 60
Power stability, % (RMS, 8 hrs): 0.05
Intensity noise, % (RMS, 20 Hz to 20 MHz): 0.4
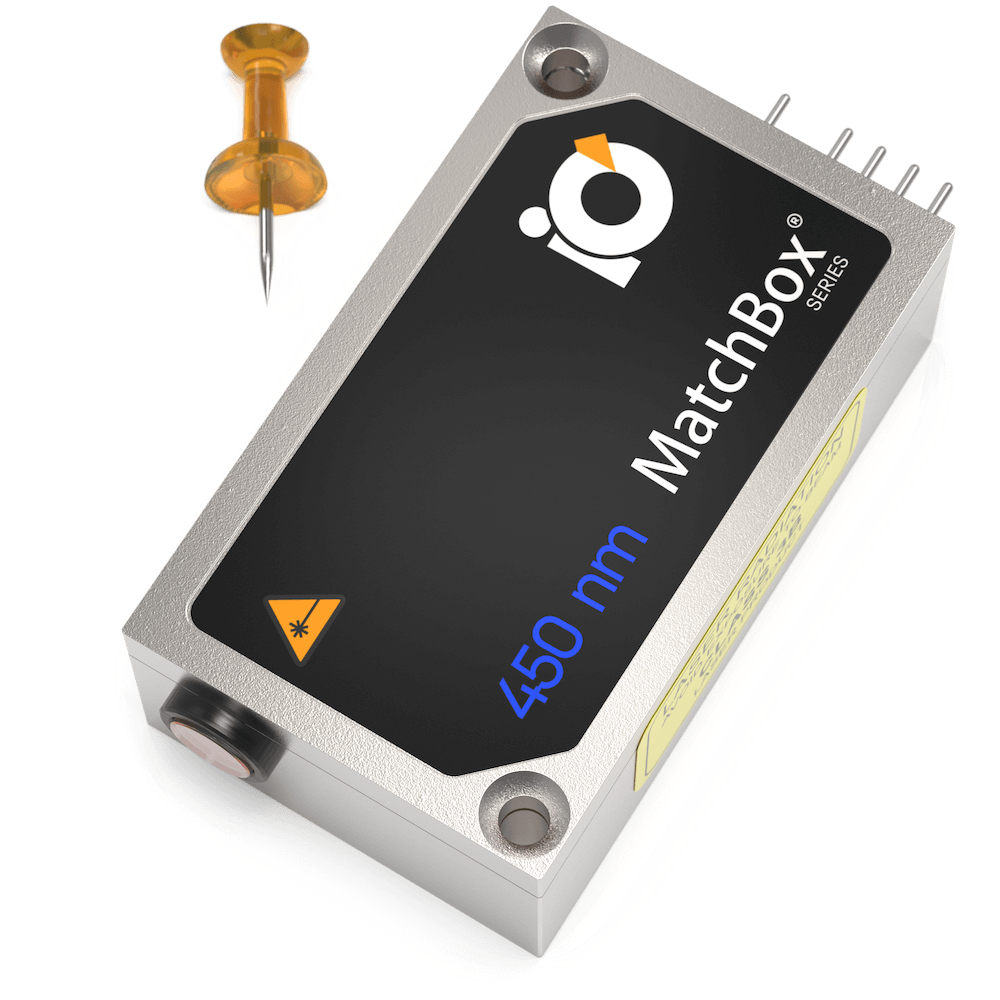
Multi-Wavelength Laser
488 nm - 40
561 nm - 50
638 nm - 130
Power stability, % (RMS, 8 hrs): 0.2
Intensity noise, % (RMS, 20 Hz to 20 MHz): 0.5
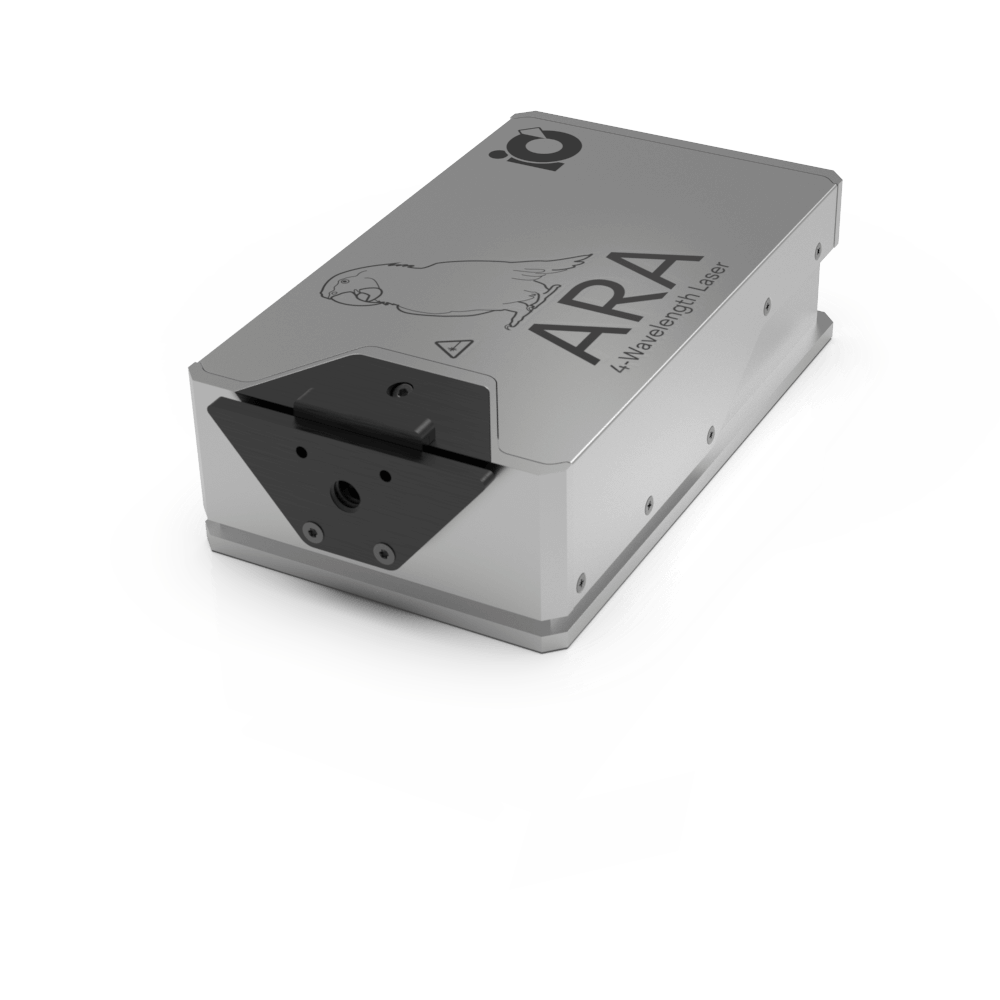
Multi-Wavelength Laser
488 nm - 40
520 nm - 80
638 nm - 130
Spectral line width FWHM, nm: 1
Power stability, % (RMS, 8 hrs): 0.2
Intensity noise, % (RMS, 20 Hz to 20 MHz): 0.5
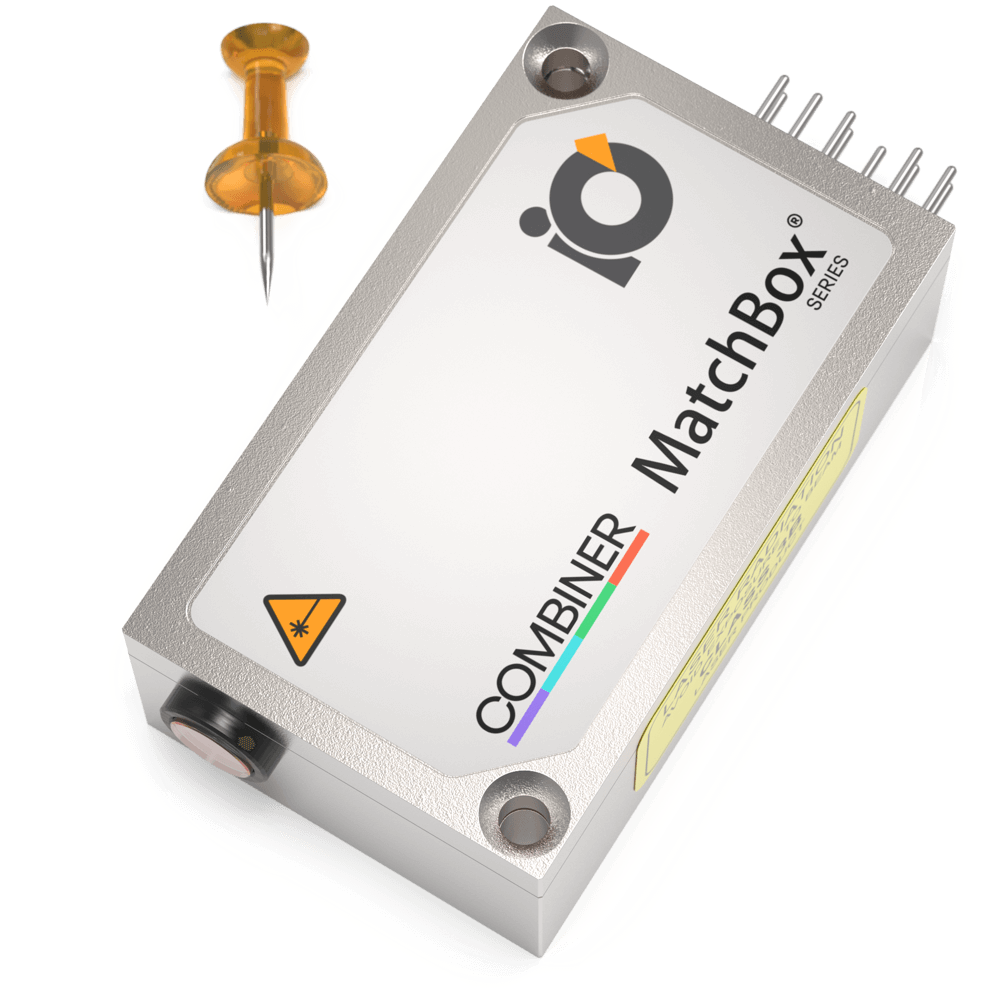
Publications
Journal of Neuroscience:
Optics Express:
Analytical Chemistry :
Advanced Functional Materials: